Higgs boson: Glimpses of the God particle
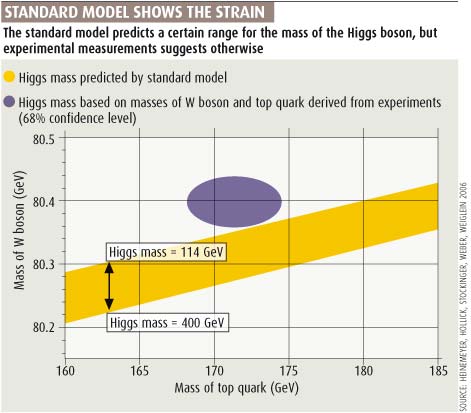
On 9 December last year, as John Conway looked at the results of his experiment, a chill ran down his neck. For 20 years he has been searching for one of the most elusive things in the universe, the Higgs boson - aka the God particle - which gives everything in the cosmos its mass. And here, buried in the debris generated by the world's largest particle smasher, were a few tantalising hints of its existence.
Conway first revealed the news of his experiment earlier this year in a blog. Experimental particle physicists are sceptics by nature, loath to claim the discovery of any new particle, let alone a particle of the Higgs's stature, and in his blog Conway dismissed hints of its existence as an aberration, just as many other supposed signs of the elusive particle have proved to be after closer examination. The tiny blips in Conway's data have so far simply refused to go away.
What's more, using data made public last week in a second blog, another team of researchers has independently seen hints of a new particle with similar mass. Both results may yet be dismissed, but the coincidence is striking, and is one that is getting physicists excited. If they have found evidence of a Higgs particle, then it points towards the existence of a universe in which each and every particle we know of has a heavier "super-partner", an arrangement of the cosmos known as supersymmetry.
The Higgs boson is infamous as the only particle predicted by the standard model of physics that remains undetected. In theory, every other particle in the universe gets its mass by interacting with an all-pervading field created by Higgs bosons. If the Higgs is discovered, the standard model could justifiably claim to be the theory that unifies everything except gravity.
But the model is creaking. Take the Higgs itself. The standard model tightly links the masses of the Higgs, the W boson (the carrier of the weak nuclear force), and the top quark (one of the fundamental constituents of matter). Experiments at the Large Electron-Positron (LEP) collider at CERN, near Geneva, in the late 1990s, and at the Tevatron, Fermilab's 6.3-kilometre-long particle accelerator at Batavia, Illinois, where Conway detected his blips, have homed in on the mass of the W boson and the top quark. If you use these measurements to calculate the mass range of the Higgs, and compare it with the standard model's predictions, you run into trouble. "The best measurements of the W and top quark mass don't agree well with the standard model," says Conway, who is based at the University of California, Davis (see Diagram).
Physicists such as John March-Russell of the University of Oxford go further. "If you ask most theorists about the Higgs, they will say it is very unlikely that we'll see just the standard model Higgs," he says. And that is what makes the hints of new particles seen by Conway and others so intriguing.
Super-partners
With the help of the Collision Detector at Fermilab (CDF) Conway's team has been searching for a more complex version of the Higgs than the standard model predicts - one that might support the supersymmetry model of the universe.
In supersymmetry, an electron has a heavier partner called the selectron, while quarks have squarks, and so on. Although none has yet been found, supersymmetry solves some niggling questions raised by the standard model. For instance, when particle physicists take the measured strengths of the electromagnetic and the weak and strong nuclear forces, and extrapolate them to the ultra-high energies of the early universe, they are supposed to unify. The idea is that in the early universe these forces were the same. To get the forces to unify at this grand unified theory (GUT) scale, the parameters of the standard model have to be tuned to an astounding precision of 1 part in 1032.
This extreme fine-tuning makes many theorists uneasy. Why should the properties of the early universe have to be so exact to give rise to the universe we have today? "It is like creating in a straitjacket," says March-Russell.
Supersymmetry, specifically a version called the minimal supersymmetric model, achieves this grand unification more naturally, with far less fine-tuning. The theory predicts five Higgs bosons of different masses, which makes the process by which the universe gets its mass more complicated than that laid out by the standard model with its single Higgs. "But very often, in the history of science, nature likes simple concepts, but it has quite complicated realisations," says March-Russell.
It's a manifestation of this complex reality that Conway's team has been probing. They are after one of the five Higgs predicted by minimal supersymmetry. Such a Higgs could be produced by the collision of protons and antiprotons at the Tevatron and some would decay into two tau leptons, which are heavier cousins of the electron. The taus decay immediately into other particles, and it is this debris the team was sifting through. Essentially, they were creating a plot which showed the mass of the particles that could give rise to two tau leptons on the x-axis, and the number of such particles on the y-axis.
Conway admits they only expected to see known particles decaying into tau leptons. But then, on that Saturday morning before Christmas, the CDF team saw the blip in their plot: signs that the Tevatron had produced a small number of some unknown particle with a mass of 160 gigaelectronvolts (GeV), which had promptly decayed to two tau leptons. "I thought maybe, just maybe, this could be the beginning of something," says Conway.
Convinced by their analysis, the entire CDF experiment team approved the data on 4 January and Conway presented it at a conference in Aspen, Colorado, a few days later. The team had found a signal which, in particle physics lingo, had a 2-sigma significance - a 1 in 50 chance of being a random fluctuation. Normally, to merit new particle status a signal must be significant to 5-sigma - where there's only a 1 in 10 million chance of it being a fluctuation.
"People were excited to see this," says Conway. But why was there so much excitement if the signal was statistically insignificant? That's because a supersymmetric Higgs at this mass is extremely plausible. "This kind of [Higgs] mass of 160 GeV is on the lower end of what we were expecting, but we are comfortable with it, in the context of supersymmetric models," says Jack Gunion, a theoretical physicist at the University of California, Davis.
He has been advocating another version of supersymmetry called next-to-minimal supersymmetry. When Gunion saw Conway's graph showing a possible Higgs with a mass of 160 GeV, he realised he only had to tune the parameters of his theory by about 1 part in 10 to explain it - an amount most physicists are willing to accept. "You can only do that in next-to-minimal supersymmetry," says Gunion. To make the minimal supersymmetry model of the universe fit, you would have to tune it to levels that would make many physicists uncomfortable, he says.
This is not the first time Gunion has used next-to-minimal supersymmetry to explain an anomalous signal. In the late 1990s, the LEP collider at CERN, which smashed electrons and positrons head-on, saw what seemed to be a new particle with a mass of 100 GeV. Again, the significance of the signal was about 2-sigma, not enough to claim a discovery. Because the signal did not sit well with a standard model Higgs, it was mostly ignored, and the LEP shut down in 2000, making it impossible to check the signal further. "It is still a big deal," says Gunion, because nobody could explain it."
But Gunion's next-to-minimal model could and does. "I claim that the model provides a simple explanation, namely that there is a Higgs at 100 GeV, and that it decayed in some extra ways that weren't expected."
That means the LEP data from the 1990s and Conway's latest findings from the CDF experiment could point to two of the five supersymmetric Higgs particles, one with a mass of 100 GeV and the other with a mass of 160 GeV. Gunion, for one, says that it is not such a stretch to think so. "These are very naturally explained in next-to-minimal supersymmetry."
First find the lepton
The story doesn't end there, however. Conway's initial analysis had given them an approximate mass for the Higgs, but there was a more accurate way to determine it.
Conway looked specifically for those tau leptons that were moving in the so-called transverse plane, which is perpendicular to the Tevatron's beam of protons and antiprotons. In particle interactions in a collider, energy should be conserved, but some energy can be emitted as neutrinos which cannot be detected directly. In the transverse plane, the detector can indirectly account for the missing energy of neutrinos with great precision. So by limiting themselves to interactions in the transverse plane, the researchers were able to accurately calculate the mass of the heavy particles that gave rise to the tau pairs, and put those heavy particles into bins of different masses. In each bin, they could explain, from known physics, what gave rise to the tau pairs. "Except in one bin," says Gunion. "And guess where that one bin is?"
It turns out that the bin is at about 160 GeV. It shows the merest hint of a new particle. "There are few events out there, right at the place where we might expect a bump," says Conway. "It is so preliminary, but it is there."
Conway's team is intrigued enough to pursue their signal. "We have got data pouring in now," says Conway. "We are going to take it to the next step." This involves doubling the statistics, increasing the sensitivity of the instruments, and even searching in other channels besides looking for tau-lepton pairs.
While increasing statistics could help verify the veracity of the signal, one particular analysis could nail the identity of the mystery particle. A supersymmetric Higgs should turn up with b-quarks, also known as bottom quarks, one of the six types of quarks. "If we see a Higgs being produced in association with b-quarks, that's a dead giveaway," says Conway. "That's the analysis we have been working towards for six to seven years now."
Meanwhile, another team led by Tomasso Dorigo of the University of Padua, Italy, has been independently analysing an entirely different set of particle interactions seen by the CDF experiment and it too has found hints of some unknown particle at 160 GeV. While the team is far from convinced that the signal is real, the coincidences are intriguing (see "Sticking with the standard model").
Markus Schumacher of the University of Siegen in Germany is also highly sceptical that the Tevatron has seen anything new. "If you look back in the history of particle physics, we have had a lot of 2-sigma effects," says Schumacher. "You have to wait until the Fermilab experiment analyses more of the data." Dorigo agrees that any claims of supersymmetry, based on the CDF data so far, are premature. "I have seen hints of new physics beyond the standard model coming and going, coming and going," he says.
Conway also remains cautious, expecting his team's own 2-sigma signal to be a fluctuation and "evaporate". If that is the case, then at least he has proved that the Tevatron collider is sensitive enough to catch glimpses of a host of other theoretical particles (see "Race you to the gluino").
But if the two teams have glimpsed a supersymmetric Higgs, then the doors to the unknown are wide open. "It's like the first few pages of a thriller," says March-Russell. "You get the first little hint that something strange is happening and that things are not quite what they seem. Then the evidence accumulates. We are turning the first few pages of this very interesting story."
Race you to the gluino
The chill felt by John Conway in December could be a foretaste of things to come. The 160-gigaelectronvolt (GeV) signal seen at the Tevatron particle collider suggests that it is capable of testing the supersymmetry model of the universe by searching for the "super-partners" of some of the known particles, and means that the race to find new particles between the Tevatron and CERN's 27-kilometre-long Large Hadron Collider (LHC), which is due to start up later this year, enters new territory.
The Tevatron is scheduled to run at full throttle until 2009, collecting data faster than ever before. By 2009, the LHC is expected to have enough data to start searching for supersymmetry. "If we were to make a discovery before the LHC after all these years and billions of dollars, that would be really amazing," says Conway.
Markus Schumacher of the University of Siegen in Germany, who works on the ATLAS detector for the LHC, knows only too well that the Tevatron could find new particles with undisputed certainty before the LHC. "There was always a race between the Tevatron and the LHC," he says. "It might well be that the Tevatron will be the first collider to see something."
That something could be not just Higgs particles, but other supersymmetric partners as well. Of course, it depends on whether next-to-minimal supersymmetry, with its modest fine-tuning, is the right description of reality. In that model, the masses of some of the super-partners should be in the range of about 300 to 400 GeV. That puts such particles in the sights of both the Tevatron and the LHC. Specifically, partners for the top quark and the gluon, namely the stop and the gluino, would be up for grabs.
Sticking with the standard model
Tomasso Dorigo of the University of Padua in Italy has put his money where his mouth is. A believer in the standard model of particle physics, Dorigo has bet his theorist friends a cool $1000 that it's the right description of reality. There's a small chance, however, that his own experiment will lose him that bet.
Last week, Dorigo's team announced the results from the CDF experiment looking at how Z bosons decay to b-quarks, a process described by the standard model of the universe. However, his team has seen, just as John Conway's team did last month, a few anomalous events at a mass of about 160 gigaelectronvolts.
If this is indeed a supersymmetric Higgs boson, then theory predicts the researchers should have recorded 100 such events based on the amount of data they have collected. According to Dorigo, the possibility that they have already done so cannot be ruled out. "There is an upward fluctuation of the data right at about that mass value, of the size one would expect from minimal supersymmetry," he says.
However, he still firmly believes that the signals his team has picked up are just noise in the data, and he's far from conceding his bet. "Extraordinary claims need extraordinary evidence," he says. "After thirty years of incredibly precise confirmations of the standard model we need a huge signal of new physics before I get convinced there is something beyond."
0 comentarios